Chapter 2. Analysis of dust mycobiota
Abstract
Broadloom dust samples were enumerated for culturable fungi from 369 homes in Wallaceburg, Ontario, Canada in winter, 1994. In total, 253 fungal taxa were identified. The taxa observed were consistent with other published reports on the fungal flora of household dust and indoor air. Taxa observed in the present study followed a Raunkiaer-type distribution, where several species accounted for the majority of observations (abundance), and the greater proportion of species documented were observed only rarely. A calculation of sampling efficiency according to Good’s Hypothesis suggested high overall sampling efficiency, averaging over 92 % of the total expected biodiversity. Association analysis based on two-way chi-square contingency resolved a number of species assemblages. These assemblages were primarily correlated to ecological specialization. The three main ecological categories that characterized the main assemblages comprised 1) phylloplane fungi; 2) soil fungi and 3) food spoilage/ xerophilic fungi. A number of smaller assemblages contained species known to be active contaminants of water damaged building materials.
Introduction
It has long been hypothesized that indoor dusts possess allergenic properties (Lucretius, 50 BCE). However, the importance of fungal materials as dust-borne allergens, was not demonstrated with fair certainty until early in the 20th century (Flood, 1931; Hopkins et al., 1930). Recently, however, there have been efforts to study household dust as an ecosystem, in an attempt to determine the characteristics of dust-borne fungi that facilitated their diversification into this relatively new habitat (Davies, 1960; Bronswijk, 1981; Bronswijk et al., 1986; Swaebly and Christensen, 1952; Verhoeff et al., 1994). It is difficult to interpret much of the existing literature in this area for two main reasons: 1) many authors provide only genus-level identifications of fungi, or employ species names that are antiquated or confused; and, 2) the lack of voucher isolates precludes the confirmation of results.
The present study is an examination of the fungal flora of household broadloom dust obtained during the course of a larger research project funded by the Canada Mortgage and Housing Corporation (CMHC) (Ottawa). This project was sought to measure various parameters of environmental exposure incurred by housing occupants, and attempted to correlate these findings to objective measures of illness. Within this context, the present study shall (1) determine the numbers and kinds of moulds present in household broadloom dust in a study site in Wallaceburg , and (2) investigate statistical associations between different mould taxa in an attempt to form hypotheses on sources and contamination pathways.
Materials and methods
Field work for this study was conducted by a private company under contract from CMHC over a period of five months starting in January, 1994. Initially, 400 households in Wallaceburg, Ontario agreed to participate in Phase I the study (see Figure 2-1).
Collection of dust samples
Vacuum cleaner bag samples of carpet dust were collected from 369 houses. Sample coverage is illustrated on the residential street map shown in Figure 2-2. These samples were supplied in sealed, 10 mL polypropylene vials which were stored at room temperature until analysis.
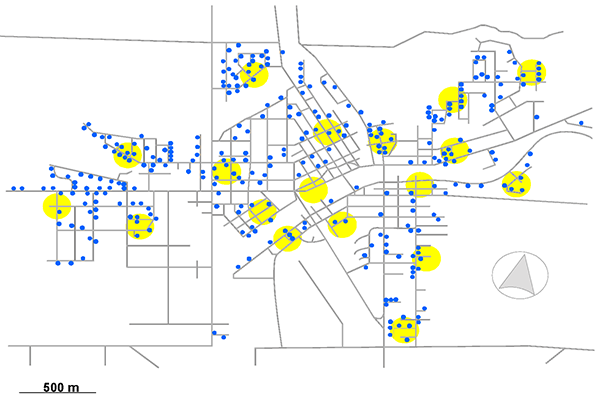
Fig. 2-2: Location of homes sampled in Wallaceburg, Ontario, Canada (blue dots). Outdoor air samples were additionally collected at a number of sites throughout the town (locations indicated by yellow circles).
Analysis of dust samples
Two subsamples of approximately 50 mg (the actual mass was recorded) were added individually to 10 mL of sterile 2% peptone broth and suspended by vortexing at medium speed. Two serial dilutions of these stock suspensions were made subsequently using an adaptation of the standard technique reviewed by Malloch (1981); the first was made by diluting 1 mL of stock suspension in 9 mL of 2% peptone broth and the second was made by diluting 1 mL of the first serial dilution in 9 mL of 2% peptone broth. Four aliquots of 1 mL each were taken from each of the two sets of dilutions and dispensed individually into polystyrene 90 mm Petri dishes (Fisher). Two Petri plates were set up in this manner for each of the two stock suspensions. Molten sterile Rose Bengal agar (RBA) (Malloch, 1981) and RBA containing 25 % glycerol (RBGA) as an osmoticant. Both media were amended with 60 ppm of chlortetracycline hydrochloride (Sigma), streptomycin sulphate (Sigma) and benzylpenicillin (penicillin-G, Sigma), were cooled to 45 °C and aseptically dispensed each into half of the Petri plate replicates at a volume of 25 mL per plate using a Pour-o-matic Petri plate filling machine (New Brunswick Scientific, New Jersey). The medium was mixed with the dilution aliquot by gently swirling the Petri plates prior to solidification. A schematic of the plating regime is provided in Figure 2-3.
After 12 to 18 hr the plates were removed from the machine, wrapped with Parafilm (Alcan) and inverted in stacks of 20 plates each. The plates were incubated under 12 hr artificial daylight at room temperature (ca. 24 °C) for 7 days. One of the three sets of dilutions averaging between 15 and 60 colonies per plate was selected for identification and enumeration. Except where one of the media used could not be enumerated at the dilution selected (due to excessively high- or low numbers of colonies or contamination) the same dilution set was enumerated for both media. This method was refined by multiple preliminary experiments. Microscopic mounts were made in either distilled water with Photoflo (Kodak) or lacto-fuchsin (Carmichael, 1955). All culture media and stock solutions were autoclaved for 20 min at 15 psi prior to use. Sterile glass and plastic-ware were used for all aseptic procedures.
Identification and isolation of cultures
Where possible, fungi were identified to the genus level directly from colonies on the Rose Bengal isolation media using well-established techniques of macroscopic and microscopic examination and standard reference works for the identification of moulds (e.g. Arx, 1970; Barnett and Hunter, 1986; Barron, 1968; Carmichael et al., 1980; Domsch et al. 1980; Ellis, 1971, 1976; Hanlin, 1990; Malloch, 1981). Many isolates were further identified to species level using appropriate monographs.
Species of Penicillium were grouped according to macroscopic and microscopic similarity, averaging five groups per house. Representatives of each of the groups with similar Penicillia were subcultured on four diagnostic media for further identification using a central-point inoculation technique modified from Pitt (1979). The Petri plates were wrapped with Parafilm, and incubated inverted under 12 hr artificial daylight at room temperature for 7 to 14 days prior to examination. The following media were employed in the identification of cultures of Penicillium to species level: Czapek’s yeast-autolysate agar (CYA) (Pitt, 1979), Creatine sucrose agar (CREA) (as modified by Frisvad, 1985), 25% Glycerol-nitrate agar (G25N) (Pitt, 1979) and Modified Leonian’s agar (MLA) (Malloch, 1981). Multiple unique isolates of Penicillium spp. were retained for subsequent use. Cultures were identified according to colonial and microscopic morphologies produced on these media and compared to the species descriptions given by Pitt (1980, 1988). Other fungi that could not be identified directly on RBA and RBGA plates were subcultured on a range of other growth media (including CYA, MLA, 2% water agar, Weitzman-Silva Hutner’s agar and Sabouraud’s glucose agar (Malloch, 1981)) and incubated in a manner similar to that used for the species identification of Penicillium cultures.
Reliability of identifications
This study involved the examination and identification of over 200,000 individual fungal colonies. Over half of these identifications were performed by the author; the balance were carried out by B.M. Koster, D. Malloch and L.J. Hutchison in addition to several contracted student assistants (see Acknowledgements). Discussions between the author and other project workers during the plating/ isolation phase suggested that the rate of misidentifications was relatively low, and typically restricted to rare or difficult taxa (e.g. Pencillium aurantiogriseum group). This speculation has been supported by retrospective examination of many retained cultures. For the purposes of the analyses presented in this chapter, all identifications were accepted as accurate and comparable; however, the reader is cautioned that the reliability of identifications may vary to some extent according to the skill of the investigator and the condition of the particular isolate examined.
Storage of cultures
Each representative isolate of Penicillium was subcultured in duplicate into 2 mL screw cap (with rubber “O”-ring) flat-bottom microcentrifuge (microculture) tubes (Sarstedt) containing 1 mL per tube of 2 % MLA. Additional representatives of Penicillium and other genera were subcultured in a similar manner pending future need. The tubes were capped and incubated under 12 hr artificial daylight at room temperature for 7 to 10 days prior to transfer to 5 °C for short-term storage. Cultures requiring long-term preservation (e.g. for use in fingerprinting) were subcultured subsequently and checked for purity. Axenic cultures were subcultured in triplicate in microculture tubes and incubated as outlined above. After colonies had grown out, cultures were aseptically overlaid with 1 mL sterile 20 % glycerol combined with 17 % skim milk as a cryoprotectant and stored at 70 °C (McGinnis and Pasarell, 1992).
Organization and analysis of data
Data were compiled using the Borland Paradox database (ver. 3.0, Borland Corp.). The database consisted of one record for each occurrence of a species on each plate examined (for both RBA and RBGA). Each record comprised 6 fields: House number; Plate identifier; Species name; Number of colonies counted; Mass of dust used in subsample (mg); and, Total number of plates of each medium (RBA or RBGA) that were examined for that house. Subsequent manipulations of the data including statistical analyses were carried out by means of specific software routines programmed and compiled by the author using QuickBasic (ver. 4.5, Microsoft Corp.).
An index of sampling efficiency (I) was calculated using Good’s Hypothesis as modified by Moore and Holdeman (Good, 1953; Moore and Holdeman, 1974), such that;
(1)
I = (1-n1/nT) × 100
where,
n1 = number of species observed once
nT = total number of observations of all species
Presence-absence (occurrence) data for taxa present in 2 % or more of samples were analysed using chi-square association analysis with Yate’s correction factor for small datasets (Greig-Smith, 1964; Kent and Coker, 1992), such that:
(2)
χ² = [n(ad-bc-n/2)²] / [(a+b)(c+d)(a+c)(b+d)]
where,
a = observed number of relevés containing “a & b”
b = observed number of relevés containing “a” only
c = observed number of relevés containing “b” only
d = observed number of relevés lacking “a & b”
n = total number of relevés examined
χ² = chi-squared statistic
The relative degree of association was summarized graphically for all combinations of taxa involved in one or more significant associations based on negative inverse logarithms of χ² values. These values were compiled into an artificial “distance matrix” in which closely-associated pairs of taxa had smaller interposing distances relative to those with lesser associations, which were separated by greater distance. A cluster analysis of this matrix was conducted using the UPGMA method in the PAUP* software package (PAUP* version 4.0b4a for 32 bit Microsoft Windows, Sinauer Associates, Inc., Sunderland, Massachusetts). It is acknowledged that as test statistics, χ² values calculated from pair-wise contingency of nominal data such as those in the present study represent multiple non-independent comparisons, and thus should not properly be analysed in un-corrected combination. It is important to note, however, that the use of chi-square values in the present application is solely a measure of the degree of association and not an assessment of the statistical significance of correlation (see Causton, 1988). Following UPGMA cluster analysis, terminal clusters of three or more closely-associated taxa were pruned from the dendrogram and re-examined graphically as 2-way matrices upon which the level of significance and polarity of association of individual χ² statistics were encoded.
Results
Species diversity and distribution
Two hundred and fifty-three fungal taxa were isolated from the 369 dust samples analysed. The proportion of house dust samples containing each taxon are summarized in Figure 2-4. A complete list of abbreviations used is given in the front matter. The distribution of fungal taxa in the study site was of a Raunkiaer-type, whereby species were distributed unevenly in abundance. In this model, most species were observed only rarely, and the majority of individuals in the population comprised relatively few species (Daubenmire, 1968; Raunkiaer, 1934).
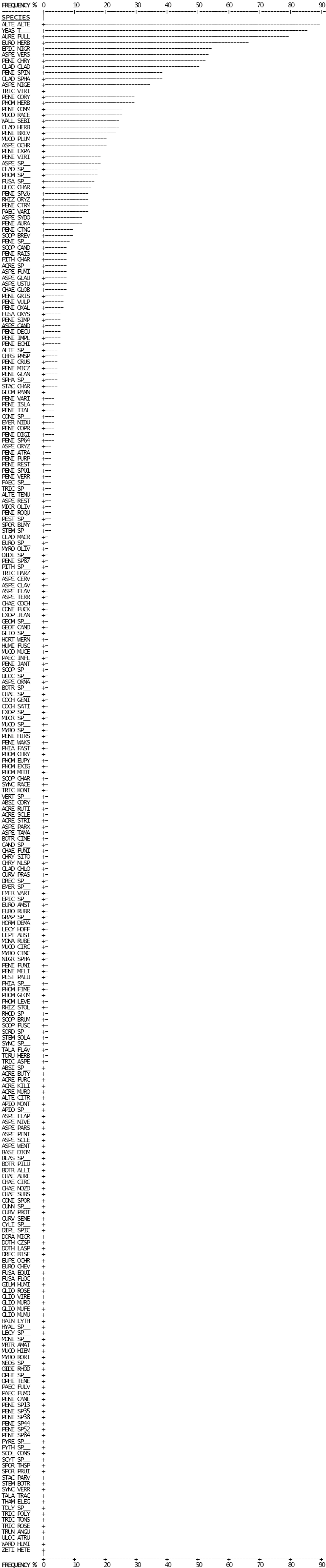
Fig. 2-4: Frequency of dustborne fungi observed in broadloom dust samples from Wallaceburg, Ontario.
Thirty-three taxa were observed in 10 % or more of samples; six of these taxa, Alternaria alternata, Aureobasidium pullulans, Eurotium herbariorum, Epicoccum nigrum, Aspergillus versicolor and Penicillium chrysogenum were present in 50 % or more of the samples analysed. Forty-three taxa were observed in 2 to 10 % of samples, while an additional 177 taxa were present in fewer than 2 % of samples. Interestingly, unidentified yeasts were observed in 85 % of samples analysed while the most commonly encountered filamentous fungus, Alternaria alternata, was observed in 89 % of samples. The most commonly occurring species of Penicillium were: P. chrysogenum (52 % of samples), P. spinulosum (39 %), P. corylophilum (29 %), P. commune (26 %) and P. brevicompactum (23 %). Most taxa observed were anamorphic species; however, a few sexual species were encountered (e.g. Eurotium herbariorum, Sordaria spp., and Eupenicillium ochrosalmonium).
Efficiency of sampling
According to Moore and Holdeman’s modification of Good’s hypothesis (Good, 1953; Moore and Holdeman, 1974), the species that were observed comprised, on average, 92.1 % (8.8 %, SD) of the total expected biodiversity in the study site. This result, in combination with the Raunkiaer-type distribution of taxa within the sample set, suggests that an increase in the number of sample sites would have only contributed to an increase in the number of rare species observed (see Figure 2-5).
Species abundance
The calculated abundance data (not presented here) showed numbers of colony-forming units (CFU) per gram of dust that ranged, on average, from 2 to 10 CFU/mg; but, species abundance occasionally was observed as high as 500 CFU/mg. Generally, yeasts and yeast-like fungi (e.g. Aureobasidium pullulans) tended to occur at greater abundances than strictly filamentous species. Due to the relatively low number of replications per sample (i.e. the number of plates examined) which varied from 4 to 8, the standard deviations of average species abundances tended to be excessive, often in excess of calculated means. For this reason, excessive outlying data would not permit trends of species associations to be resolved using canonical correspondence analysis (Braak, 1992). Species that occurred at a low frequency (i.e. in fewer than 2 % of samples) tended to skew the χ² statistic artifactually towards significance. For this reason, rare species were eliminated from this analysis. The results of significant χ² associations are shown in trellis-diagram format in Figure 2-6. The 52 pairings of species represent the significant associations (a = .05, df = 1) that resulted from the inspection of 3,003 pairings (see Appendix B). The first 21 couplets were highly significant (a=.001, see Appendix B).

Figure 2-6: Trellis diagram summarizing significant associations of dustborne taxa.. Positive associations are indicated by † (α=0.05) ‡ (α=0.001). Negative associations are indicated by -(α=0.05) and = (α=0.001).
Association analysis
The 52 associations of significance involved forty-seven taxa (including a category of unidentified yeasts); forty-three of these associations were positive correlations while the remainder (9) were negatively associated. Interestingly, Cladosporium herbarum was a partner in two thirds of the negative associations.
The results of this association analysis are presented as a dendrogram based on average distance by an inverse logarithmic transformation of the χ² value. Overall, 14 clusters of more than two taxa were observed (see Figure 2-7).

Figure 2-7: Cluster analysis based on associations between dustborne fungi determine by pair-wise Chi-square contingency.
These terminal clusters of 3 or more taxa are shown in Figure 2-8, and their polarity is indicated. Each terminal branch is shown as a matrix, in which the positive or negative nature of the correlation is shown by a shaded circle at each node. Following is a summary of these terminal clusters:
Fig 2-8: Significant associations between taxa in significant clusters (> 2 taxa).
Cluster a) An unidentified species of Paecilomyces was positively correlated to Penicillium coprophilum (see Figure 2-8a). This pair was negatively correlated to Trichoderma sp. and Penicillium italicum (the latter pair also showed a negative correlation).
Cluster b) A similar arrangement was observed in Figure 2-8 cluster b, in which Coniothyrium sp. was positively correlated to Penicillium vulpinum yet this pair showed negative correlation to Emericella nidulans and Penicillium restrictum (the latter two also showed negative correlation).
Cluster c) Stachybotrys chartarum was negatively correlated to the positively-associated pair Penicillium implicatum and Penicillium miczynskii (see Figure 2-8c).
Cluster d) Cluster d (Figure 2-8d) showed positive associations between Penicillium atramentosum, Penicillium sp. #26, Aspergillus glaucus, Alternaria sp. and Penicillium corylophilum. Within this assemblage, however, a negative association was observed between Alternaria sp. and Penicillium sp. #26.
Cluster e) Geomyces pannorus was positively associated with Paecilomyces variotii (see Figure 2-8e). An unidentified species of Aspergillus showed a negative correlation to the former, and a positive correlation to the latter. Collectively, this group was negatively associated with Scopulariopsis candida.
Cluster f) Fusarium sp. in cluster f (Figure 2-8f) was positively allied with Penicillium griseofulvum and Penicillium digitatum (the latter two were negatively associated). This group was negatively associated with the positively correlated pair of Penicillium purpurogenum and an un-named Penicillium species.
Cluster g) The species negatively-associated assemblage in cluster g (Figure 2-8g) comprised Aspergillus sydowii, Penicillium islandicum and Penicillium decumbens.
Cluster h) The three taxa Chrysosporium sp., Cladosporium sp., Pithomyces chartarum and uncategorized yeast were positively associated in cluster h (Figure 2-8h). This group was negatively correlated to Cladosporium herbarum.
Cluster i) Mucor racemosus was positively correlated with Penicillium viridicatum, which was further positively correlated to Penicillium citrinum (see Figure 2-8i). However, Penicillium citrinum showed a strongly negative correlation to Mucor racemosus. As well, an unidentified species of Phoma showed negative correlations to all taxa in this cluster (Figure 2-8i).
Cluster j) Penicillium chrysogenum was negatively correlated with Penicillium brevicompactum, yet these two taxa were positively correlated with Aspergillus flavus-oryzae complex (see Figure 2-8j). Within this assemblage, Penicillium verrucosum showed a positive association with Aspergillus flavus-oryzae complex and Penicillium brevicompactum and a strongly negative association with Penicillium chrysogenum.
Cluster k) The xerophilic fungi Aspergillus versicolor, Eurotium herbariorum and Wallemia sebi formed a positively correlated assemblage (see Figure 2-8k).
Cluster l) There were positive associations between the typical phylloplane fungi, Alternaria alternata, Aureobasidium pullulans, Cladosporium cladosporioides, and Epicoccum nigrum (see Figure 2-8l). This assemblage tended to be negatively associated with Penicillium aurantiogriseum.
Cluster m) The phylloplane taxon Ulocladium chartarum was positively associated with Cladosporium sphaerospermum and Phoma herbarum. This assemblage was collectively negatively associated with the xerophilic fungus Aspergillus ustus group (see Figure 2-8m).
Cluster n) Similar to Cluster k (As. versicolor, Eu. herbariorum and W. sebi), Aspergillus niger group was positively associated with Penicillium spinulosum and Penicillium simplicissimum (see Figure 2-8n).
The remaining couplet assemblages are summarized in Table 2-1. Ten of these associations were positive and one was negative (Acremonium sp. with Penicillium echinulatum). Of the positive associations, several are of interest. In particular, the soil fungi Aspergillus candidus and Aspergillus fumigatus were associated. Aspergillus ochraceus showed and association with Penicillium variabile. Both of these taxa are common indoor contaminants on damp building materials (Adan and Samson, 1994). Similarly, Chaetomium globosum showed an association with Penicillium crustosum. These taxa are common colonists of very wet gypsum wall board. Mucor plumbeus was correlated with Rhizopus oryzae. Both of these taxa are rapid colonists of substrates under high disturbance, particularly in the presence of simple carbohydrates (e.g. such as decaying fruits) (Samson and Reenen-Hoekstra, 1988). Penicillium brevicompactum showed a positive association with Penicillium commune. These Penicillia occur frequently on indoor finishes, particularly wall paper or drywall which incurs intermittent wetting. The soil fungi Penicillium citreonigrum and Trichoderma viride were positively associated. For comparative purposes, the houses which were observed to have active mould growth on indoor surfaces are summarized in Table 2-2.
Table 2-2: Houses with visible mould growth observed
DISCUSSION
The most commonly occurring species found in the present study are consistent with reports on the species composition of household dust by other authors (Bronswijk, 1981; Calvo et al., 1982; Davies, 1960; Gravesen, 1978; Hamada and Yamada, 1991; Ishii et al., 1979; Lustgraaf and Bronswijk, 1977; Mallea et al., 1982; Saad and El-Gindy, 1990; Schober, 1991; Swaebly and Christensen, 1952; Wickman et al., 1992). The species assemblages of dust microfungi observed in this study comprised a mixture of ecologically homogeneous groups:
- Phylloplane (leaf surface) moulds (e.g. Alternaria alternata, Aureobasidium pullulans, Cladosporium cladosporioides and Epicoccum nigrum), all of which were negatively correlated to Penicillium aurantiogriseum, a typical xerophilic food spoilage fungus.
- Xerophilic moulds typically associated with food spoilage such as Aspergillus versicolor group, Eurotium herbariorum and Wallemia sebi (see Pitt and Hocking, 1985; Samson and Reenen-Hoekstra, 1988).
- Soilborne fungi such as Trichoderma viride and Penicillium citreonigrum (see Barron, 1968; Domsch et al., 1980). The existence of these distinct species assemblages was corroborated by chi-square association analysis.
Besides the groups with obvious ecological similarity, several species assemblages were noted that contained taxa notable as contaminants of water-damaged building materials or finishes (e.g. Aspergillus versicolor, As. ustus, Chaetomium globosum, Penicillium aurantiogriseum, Pe. brevicompactum, Pe. chrysogenum and Stachybotrys chartarum group). Often these taxa (e.g. As ustus, Pe. aurantiogriseum, etc.) were negatively correlated to clusters of taxa from defined ecological groups discussed above. It is likely that these taxa are true household residents, as agents of structural contamination or growing in dust following episodic wetting.
The results of this study showed disproportionately high viable levels of small-spored fungi, such as Aspergillus or Penicillium (data presented in Appendix A). This observation is consistent with those of other workers (e.g. Flannigan and Miller, 1994), who have suggested that additional factors besides proliferation in situ may lead to accumulation of these spores in dust in homes absent of indicators of mould problems (e.g. in good repair, clean and free from water damage) and objective measurements demonstrate a high standard of IAQ (Flannigan and Miller, 1994; Scott et al., 1999a). Scott and co-workers (1999a) postulated that dilute levels of spores of these taxa from outdoors might enter houses passively and accumulate in dust, either because of differentially long viability period of spores of these species or through mechanical means. The large component of phylloplane fungi in house dust suggests that, although these fungi may in some cases become resident in homes, their collective occurrence in dust is a function of their abundance in outdoor air; thus, in house dust, the accumulation of propagules of these fungi likely results from the settling of spores from ambient air. The fact that many small spored taxa are often well-represented in carpet dust may relate to the tendency of these spore types to be produced by phialidic species, many of which produce spores prolifically on compound conidiophores with the number of spores per conidiophore greatly in excess of many common, non-phialidic phylloplane moulds (e.g. Alternaria, Cladosporium). Differences in viability over time between these two spore types may also be important. For instance, the relatively thick-walled nature of these typically phylloplane spores intuitively suggests an adaptation to increased spore viability; however, Sussman (1968) documented the disproportionate longevity of small, thin-walled globose spores such as Aspergillus and Penicillium, relative to larger, thicker walled spores of phylloplane moulds. Despite the lack of input of xerophilic species into the indoor environment from outdoors during winter months while windows remain closed to conserve heat, a stable reservoir of propagules of phylloplane moulds remains in the biological “memory” of the carpet. Flannigan and Miller (1994) recognized the longevity of Aspergillus and Penicillium spores in household dust, and suggested that this property may permit the use of dust as a long-term spore trap which, by analysis, could reveal transient bursts of airborne spores of these taxa over time. However, Verhoeff and co-workers (1994) found only a weak correlation between houses that had objectively demonstrable mould problems and a substantial change in the dust mycoflora, suggesting that dust analysis may not be a reliable predictor of indoor contamination.
The viability of the mycoflora was followed over three years for dust samples from selected Wallaceburg stored under dry conditions. Fifteen dust samples (indicated in bold in Table 2-2) in follow-up testing showed the complete dying off of all taxa except Aureobasidium pullulans and Penicillium spp. These results confirmed the observation of Sussman (1968) that spores of species of Aspergillus and Penicillium remain viable for a much longer period of time compared to most phylloplane fungi under our storage conditions (e.g. Alternaria, Cladosporium, Epicoccum and Ulocladium and support the hypothesis that the culture-presence of high numbers of Aspergillus and Penicillium relative to phylloplane taxa from household dust may be an artifact of the longer period of spore viability of the former group. Davies (1960) proposed that mould colonization of house dust required at least one of several conditions to be met: 1) the direct observation of conidiophores in the dust; 2) the ability to culture particular fungi from dust-borne mycelial fragments; and, 3) an abundance of a particular fungus in dilution plates that significantly exceeds the representation of that same species in control samples. These principals are supported by the present study.
I hypothesize that various routine mechanical processes within houses permit the accumulation of fungal spores. Since spores of Aspergillus and Penicillium are typically in the size range of 2-5 µm in diameter it is possible that these particles may penetrate inefficient filters, such as dust bags in portable vacuum cleaners or filters in forced-air heating systems, and thus, not be efficiently removed from the indoor environment by these processes (Stetzenbach et al., 1999). This being the case, a properly installed, externally-vented central vacuum cleaner may help to reduce the accumulation of indoor mould spores.
The negative correlation of Cladosporium herbarum with a number of other, presumably resident carpet dust fungi may result from its particular abundance in outdoor air during early spring (Abdel-Hafez et al., 1986, 1993; Ripe, 1962). Additional examinations of outdoor air samples by the author have shown that C. herbarum is typically the most abundant species of Cladosporium isolated from outdoor air from early spring through summer in Toronto. This species remained relatively uncommon in dust samples until late spring, at which point, the colony counts of C. herbarum became sufficiently high that higher orders of dilution were used in order to maintain overall counting efficiency (data not shown). As such, the occurrences of other species that remained present at roughly constant background levels were displaced by the high input of C. herbarum and proportionally were observed to decrease.
Soilborne fungi occur only at very low levels in indoor air, and their presence in indoor environments is mostly limited to the soil of indoor, potted ornamental plants (Summerbell et al., 1992). The results of the present study indicate that many common soilborne taxa occur frequently in carpet dust as well. This may result from the commonplace North American practice of wearing footwear in the house, a custom uncommon in most parts of Europe and Asia.
The indices of coverage that were calculated using Good’s hypothesis showed that in all but a few cases (e.g. house numbers 137, 288 and 291) the sampling technique employed was adequate to detect close to the total number of species expected in each dust sample (see Appendix C). Nevertheless, the number of replications that would be required to establish statistical confidence in abundance data is prohibitively large in a study of this magnitude. Even so, the interpretation of abundance data is complicated by the tendency of heavily sporulating fungi such as Aspergillus, Penicillium and fungi exhibiting yeast-like growth forms to be over-represented in surveys employing serial dilution techniques.
Conclusions
The fungal flora of broadloom-bound house dust consists of a mixture of active and passive residents. The passive occupants of carpet substrates are mainly allochthonous, and arise from ecologically diverse habitats, mostly outside of the indoor environment. The main sources of allochthonous indoor moulds are 1) the phylloplane; 2) soil and 3) xerophilic fungal food-spoilage. The accumulation of spores of these fungi within broadloom dust is a result of multiple mechanical processes operating within the indoor environment. These processes may include the propagation of phylloplane spores indoors on air currents and clothing and the movement of soil-borne fungi indoor on footwear and by pets.
The small-spored nature of many xerophilic food spoilage fungi may favor their passage through inefficient filters, such as on vacuum cleaners and forced air furnaces. This likelihood, coupled with the differentially greater longevity relative to phylloplane taxa may facilitate their artifactual accumulation within broadloom over time. However, many of the taxa common to any of these groupings may undergo active growth in the indoor environment under suitable conditions, however, the primary sources of inoculum for these fungi are allochthonous. The active residents of broadloom dust are mostly restricted to a small subset of the total fungal biodiversity contained within this substrate. These species proliferate within broadloom dust (autochthonous), and are similarly often responsible for fungal disfigurement of other indoor finishes and building elements under conditions of water accumulation (e.g. As versicolor, W. sebi).